The Science of Brakes
300mm of steel, a rubber hose and some fluid is enough to bring 200 kilos of bike to a standstill from 200mph in 10 seconds. Here's how
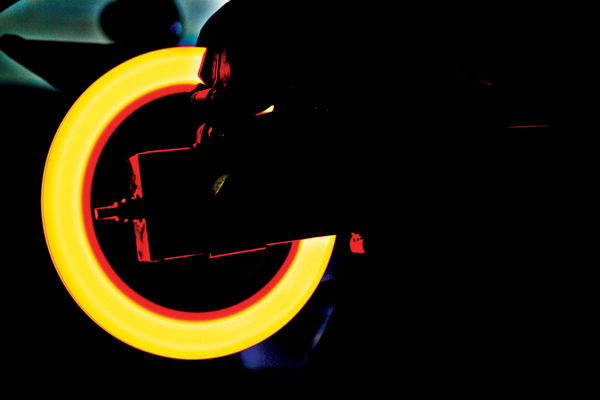
Brakes have been accelerating, at least in terms of their technology, in the past decade. Growing pistons, sprouting new mounting systems, gaining power assistance, and even some cast-in-steel certainties you could always depend on, like discs being round, have started to look wobbly. Or wavy.
The impressive reductions in mass of most manufacturers' bikes in the past few years might have taken some pressure off what stoppers have to cope with, if only riders weren't busy getting fatter and spoiling it.
But the main forces driving the advance are improvements in tyre technology and increasing top speeds. More grip from road rubber, pioneered by more grip being found in race tyres, means brakes have to be stronger to be able to exploit it. And they need to do so in as easy-to-control a manner as possible if they're to be of any use.
Higher top speeds (and on MotoGP bikes we're now talking in terms of 210mph-plus regularly, while many road bikes are capable of at least 180mph) carry a double whammy, or more precisely, a whammy squared. A brake's job, at its most fundamental level, is to convert your kinetic energy - the energy you have due to your speed - into heat. The faster it does that, the more rapidly you slow down.
What makes its job so demanding is that your kinetic energy increases in proportion to the square of your speed, so a bike at 120mph has accrued four times the kinetic energy it had at 60mph. It's not quite true to say the brake therefore has to shed exactly four times as much heat at twice the speed because wind resistance is on your side for once. This also increases with the square of your speed, so it's trying four times harder to slow you down at 120mph as at 60mph and the brakes get a bit of help.
But not enough to make a big difference - the square of your speed is the dominant factor, and you can be sure that the kinetic energy of a fully fuelled Hayabusa with a 15-stone rider at 200mph has to be converted into an awful lot of heat to lose through the brakes when it's slowing to a standstill. We wouldn't recommend sticking your tongue on the discs straight afterwards to hear how loud it hisses! In the dark, they'll be a glowing, red-hot 800°C. They can get up to 400°C even in normal road use, so if you value your taste buds, disc-licking is probably best restricted to before you set off. If you really must.
You don't have to use the brakes of course, you could coast to a stop from 200mph eventually. But still the same amount of energy would have to be lost, which happens as heat in the tyres and bearings, a small
fraction as noise, and kinetic energy (plus a tiny amount of heat) given away to the air as turbulence. It just takes longer. What brakes do is shortcut the process, grabbing big buckets of your kinetic energy and gobbing it out as heat as fast as they can, depending on how hard you're pulling the lever.
And that's the bit that matters, what happens when you pull the lever. Years ago, this would usually operate two shoes inside a drum via a cable. But drum brakes are limited because the heat is generated inside the wheel, away from the cooling airflow, and the length and curve of brake shoes means very high pressures can't be applied without distorting them. So disc brakes were invented.
The friction surface where the heat is generated is out in the open, the disc and caliper are simpler lumps of metal that suffer less from heat distortion and because brake pads are small, chunky, square-ish things, much higher pressures can be applied to them than shoes, resulting in an increase in friction and braking force.
In all but a handful of cable-operated oddities, those forces are applied by hydraulics, a very useful way of translating and magnifying a force from somewhere convenient to somewhere awkward and mobile, like from a handlebar to a caliper on a bouncing fork stanchion.
The advantage starts when you squeeze the lever, as this gives you immediate, er... leverage, over the brake's master cylinder of about four to one. Move the lever 20mm where you're squeezing it and it pushes a piston into the master cylinder by 5mm, but with a four times larger force than you're applying. If that piston has a 10mm diameter, it displaces about 0.4cc of brake fluid into the brake hoses. The fluid doesn't compress and decent hoses won't expand significantly, so at the other end 0.4cc is displaced into the only place it can go - behind the piston in the caliper that pushes the brake pad against the disc. Let's say this piston has a 40mm diameter, in which case it only needs to slide forward 0.31mm to accommodate this extra 0.4cc.
So, a 5mm movement of the piston at one end produces just 0.31mm of movement at the other, 16 times less movement but with 16 times more force. If you now factor in your brake lever gains, you've turned 20mm finger movement into 0.31mm piston movement and multiplied the force a further four times, a total of 64 times. You have the power...
The small amount of movement of the caliper piston is not a problem, as brake pads are brushing against a disc's surface anyway. The consequence is the pads can be squeezed against the disc with colossal force, the friction rises dramatically, loads of heat is generated and the bike slows down.
This is the underlying principle, what comes next is refining it to make it work more effectively. And this is where compromises come into play, as with all engineering. The first and most obvious compromise is calipers and discs add weight to exactly the area it's least wanted: the unsprung mass of a wheel assembly and suspension. More mass here adversely affects ride quality and grip and, worse still, brake discs add gyroscopic effects that slow steering speed (although they do improve stability), as well as increasing the rotational inertia of a wheel. And ironically, that impairs braking as well as acceleration.
HOW TO BED IN NEW DISCS AND PADS
Brakes perform best and last longest if properly treated - run in - when new. New pads bed in best on old discs, and likewise new discs are best bedded in with old pads, so try not to change both at once. On a brand new bike, the brake bedding in routine should be extended by around 50%.
With new pads, the aim is to boil off the resin's gases and avoid green fade or overheating the pads and causing them to glaze over, which ruins their performance. This means using them gently with no long periods of braking for 100 miles.
It also helps when you fit new pads to cross-hatch your brake discs lightly with a rotary 220 grit sander on an electric drill, to bust the discs' glaze.
Discs need to be tempered, a heating and cooling cycle that stabilises the metal structurally, making the discs more resistant to warping and wear. Do this by braking with medium pressure from 60mph to 30mph, releasing the brakes then accelerating back up to 60mph. Don't stop as it's important to maintain a flow of cooling air. After two minutes, repeat the process 10 times. Then stop to let the discs cool completely before running through another 10 cycles, using harder braking. Three or four of these 10-cycle sessions will temper your discs, but avoid very hard use for 500 miles.
Brakes cont.
This is decision time when specifying the disc diameter. A larger diameter disc (which you'll also see referred to as a rotor) will increase your braking power for simple leverage reasons that you'll understand if you imagine trying to stop a spinning cycle wheel. Grab it at the tyre, a long way from the axle, you have plenty of leverage and it's relatively easy to stop. Now try to stop it by grabbing the spokes near the hub, and you'll struggle as you don't have much leverage. In the same way, large diameter discs mean more braking power, but as they're bigger they also weigh more and increase the gyroscopic forces because of their increased diameter. The first compromise then is between stopping power and other chassis requirements.
You can make the disc thinner to shed weight, but thinner discs are more prone to distorting and warping with heat. They also need to cope with some very large forces, so you don't want them so thin they just break or crack when used in anger.
Another solution is to make the disc narrower when viewed from the side, so its inner diameter is closer to its outer diameter. The disc becomes usefully lighter, but the problem is you would have to make the brake pads smaller, reducing friction and negating any other gains. The answer here is to make the brake pads longer and thinner to maintain the total area of contact with the disc. But brake caliper pistons have to be round, so to keep the pressure even across the pad, the solution is to have two pistons operating on the one pad, one at each end. With a pad and two pistons on each side of the disc, you have a four-piston caliper, as used on most self-respecting sportsbikes.
You can extend the concept a stage further, making the disc even narrower radially and the brake pads even longer and thinner, so much so they now need three pistons in a row to keep the pressure on them reasonably even, which leads to the six-piston caliper.
The benefit is not just in weight loss. The average force applied by a brake pad acts at its centre, and on a narrow pad this will be further out from the wheel's centre than on a wide one, assuming both are fitted to discs with the same outside diameter. Which means using longer, narrower pads has the same effect as using a bigger diameter disc, i.e., more braking power.
A further refinement on four- and six-piston calipers is differential piston sizes. Under braking, the leading edge of the pad tends to be dragged onto the disc surface in what's often called a self-servo action, while the back edge wants to move away from it, as if the whole pad is trying to rotate against the disc like a gear wheel. So to compensate and maintain an even pressure along the length of the pad (which improves wear characteristics and feel), the piston at the front of the pad is smaller than the rear one, in order to exert a smaller force.
The self-servo action also fractionally delays the release of the pads from the disc, when you let go of the lever. This is countered on some race systems (e.g. Brembos on 998R and 999R Ducatis) by using four pads in four-piston calipers, two on each side of the disc. These smaller pads have a reduced servo effect compared with one long one (yet more leverage in action...), so they release more quickly and progressively. Just what you need when you're scrubbing off speed with the front as you're feathering the brake deep into a turn while diving inside Noriyuki Haga...
The relentless fight of every engineer though is not so much against physics, but accountants. And accurately machining six pistons and bores is a costly business - differential ones even more so. Even four-piston calipers are something of a luxury, which is why the floating caliper exists.
So far we've assumed a caliper is a fixed lump of metal with holes bored in it for the pistons to slide out of on either side of the disc, called an opposed-piston or fixed caliper. But many bikes are fitted instead with calipers that aren't fixed. The most basic type will have one piston only, and instead of being mounted rigidly it can slide on a pair of pins. As the piston forces its pad against the disc, so it's also trying to push the caliper body away from the disc. An extension of the caliper reaches over the disc and cradles a pad on the far side. As the caliper body slides away from the disc the opposing pad is squeezed up against it. With everything clean, greased and working properly the pressure applied by both pads is the same.
Floating caliper design too can be improved in the same way as opposed-piston (or fixed) ones, by using a pair of pistons side by side bearing on a longer pad, allowing a narrower, lighter disc to be fitted. At first glance, this looks indistinguishable from an opposed four-piston caliper (which is one of the reasons for doing this), but a closer inspection reveals the inner pad simply has metal arms behind it rather than pistons. Like for like, floating calipers are cheaper than opposed-piston ones, but floating twin-piston ones cost more to manufacture than opposed twin-piston calipers (with one piston on each side of the disc). The justification is they're slimmer, the disc is lighter and - not to be sneered at - they look better.
The main advantage of fixed calipers is exactly that: they're fixed, so less of the effort you expend at the handlebar lever goes into moving or distorting components other than the pads themselves. That's also why steel braided brake hoses improve feedback: standard rubber hoses expand a small amount as the hydraulic pressure in them increases, which means you're squeezing harder at the lever without the braking force increasing proportionally. A worst case would be with knackered old rubber hoses where the pressure in the system increases as you brake, until a point is reached where further squeezing of the lever simply makes the hoses balloon out without any useful increase in pressure at the brake pads. It does happen.
Braided hoses expand much less than even new rubber hoses, so the relationship between brake lever and pad pressure is more direct. But the hydraulic pressure and forces in brake systems are so large, even the caliper bodies themselves distort. Cheaper fixed calipers are manufactured as two halves then bolted together, but the best ones are machined (awkwardly...) from single, solid castings of aluminium (or even magnesium on competition machinery), so the potential for distortion is reduced.
Which brings us to radially-mounted calipers. Traditionally, brake calipers have been mounted at one end of their body only, which means they can stick out a long way from a fork with nothing but their own stiffness to stop them waggling about. But this new fashion is to support them at both ends of their bodies on two arms cast into the fork body, aligned radially from the wheel centre. It's an obvious and not especially expensive advance on older brake designs (the current price premium is mostly fashion led), but as it reduces the amount the brake caliper can flex in extreme conditions, it's another way of ensuring the maximum amount of lever pressure is converted directly into brake pad movement. Better feedback and control, in other words.
If floating calipers are cheapskate, floating discs are a good thing. Steel is used for discs because it wears well and copes with the loads and heat effectively, but it's heavy. So the steel rotor section is usually mounted on a lighter aluminium carrier, which in turn is bolted to the wheel hub. The problem is aluminium expands more than steel when heated, so the two are separated by 'buttons' which allow
differential expansion.
Road bikes mostly have semi-floating discs, which only means the buttons are quite a tight fit, but racers will have fully floating ones, where the disc can be rattled side to side (when it's cold) on the carrier. This allows for the greater expansion of hotter race brakes but isn't suited to road bikes, as with prolonged non-use of the brakes a rattling disc can gradually push the caliper pistons back. Then when you do want to stop you might need to pump the lever several times to return them to the disc face, disconcerting when you're trying to stand a bike on its nose to avoid T-boning a myopic Ford Sierra driver who's just pulled out in front of you.
HOW BRAKES EVOLVED...
- The drum brake works with two shoes in a hub, but distortion is a problem and heat is confined to a closed area.
- The disc brake has better cooling properties and produces better friction and braking forces.
- Radial brakes support the calipers at both end of their body to reduce flex under hard braking.